Article Sidebar
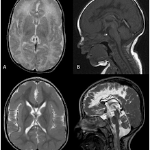
Downloads
Background
Brain malformations comprise a wide spectrum of entities that result from environmental or genetic factors interfering with normal brain development. This manuscript focuses primarily on conditions affecting the development of the cerebral cortex – malformations of cortical development (MCD), but since MCD are almost always associated with other abnormalities of the central nervous system (CNS), including white matter, corpus callosum, basal ganglia, brainstem, cerebellum, and/or cerebellar vermis, the reader is urged to always evaluate the entire brain MRI when confronted with a malformation affecting the cortex or any other brain structure.
Although the optimal classification of MCD would be based on the pathways and mechanism of protein action, with variations based on how a specific gene mutation alters protein function in the affected pathway, our current understanding of pathways and mechanisms of protein action is not yet adequate enough to classify disorders on this basis. The 2012 update on the classification of MCD therefore remains the most valuable framework to orient clinicians for the work-up of patients with MCD phenotypes[1]. In this framework, the first group of disorders is associated with abnormal neuronal and glial proliferation or apoptosis and includes severe congenital microcephaly, megalencephaly, and cortical dysgenesis or dysplasia with or without neoplasia. The second group of malformations results from abnormal neuronal migration and includes periventricular heterotopia, lissencephaly, subcortical heterotopia, and cobblestone malformation. The third group of malformations results from abnormal postmigrational development and includes polymicrogyria, postmigrational developmental microcephaly, focal cortical dysplasia without dysmorphic neurons, and cortical dysgenesis secondary to metabolic disorders[1].
This paper will highlight the added value of detailed clinical phenotyping across all settings, be it wealthy or poor, before or after an etiological diagnosis of MCD is made. In high income countries, one of the first steps in the diagnostic process for a child presenting with developmental delay, epilepsy, microcephaly, megalencephaly or cerebral palsy, will be the performance of a brain MRI. When a brain malformation is detected on imaging, a blood sample will be sent off for next generation sequencing (NGS) studies. Although the yield of NGS studies in MCD has not yet been studied in detail, studies in rare diseases or pediatric neurology in general indicate that a conclusive diagnosis can be reached in 30 to 40% of patients[2 3 4]. This still leaves almost two thirds of patients without diagnosis and hampers genetic counselling of relatives at risk. Further efforts to collect detailed phenotypic data on the patient, perform segregation studies in the family as well as functional studies, and/or identify additional families through national and/or international collaboration may further increase the number of conclusive answers for many families. When availability of NGS studies is limited, careful clinical phenotyping remains the basis for diagnostic assessment and assists the clinician in selecting those investigations which might yield the most valuable information, in order to provide optimal care to the patient and his/her family.
When evaluating a patient with MCD, the following steps might be useful to consider
Clinical Vignette 1
The proband was born at 38 weeks gestation as the first child of healthy consanguineous parents. The pregnancy was complicated by intra-uterine growth retardation. Head circumference at birth was 31cm (-2SD), birth weight 2179g, and height 44cm. Clinical examination revealed white cataract and microcornea, facial hypertrichosis, abnormal genitalia with small scrotum and cryptorchidism, adducted thumbs and rocker bottom feet. The child presented with severe hypotonia at birth, and later developed bilateral spastic cerebral palsy and severe intellectual disability. Brain MRI performed shortly after birth showed bilateral polymicrogyria predominantly over the frontal regions (Figure 1, A-B). MRI at the age of three illustrated the changing aspect of polymicrogyria after completion of myelination (Figure 1, C-D). Based on the presence of microcephaly, frontal predominant polymicrogyria, cataract and microcornea, and abnormal genitalia, a clinical diagnosis of Warburg Micro syndrome was made and targeted gene sequencing of the RAB3GAP1 gene revealed a homozygous c.52A>C (p.Thr18Pro) mutation in this child, with both parents being heterozygous carriers. The clinical phenotype and genetic basis of Warburg Micro and Martsolf syndrome have been reviewed in detail by Handley et al. in 2013[5].
This case illustrates how careful clinical examination may enable the clinical diagnosis of a specific syndrome encompassing MCD and may assist with the diagnostic work-up.
Figure 1.Figure 1Brain MRI at the age of two weeks (Panels A-B) and three years (Panels C-D) of a patient with a homozygous missense mutation in RAB3GAP1 showing bilateral frontoparietal polymicrogyria (Panels A and C), global reduction of white matter (Panel C), thin corpus callosum, and hypoplasia of brainstem and cerebellar vermis (Panels B and D).
Clinical Vignettes 2 and 3
The proband was born at 35 weeks gestation as the second child of a healthy non-consanguineous couple. She was transferred to the neonatal intensive care unit for apnoea episodes and dysmorphic features, including a large anterior fontanel, brachycephaly, a short broad neck, clitoromegaly and clubfeet. She had fluctuating blood sugar levels and elevated liver enzymes with AST and ALT up to five times upper normal levels. Brain MRI at age 19 days showed abnormal cerebral and cerebellar white matter, polymicrogyric-like cortex most severe in the perisylvian and perirolandic regions, and germinolytic cysts (Figure 2). The combination of clinical and biochemical findings led to a working hypothesis of a peroxysomal disorder, which was confirmed based on a liver biopsy.
Figure 2.Figure 2Brain MRI at age 19 days of a patient with Zellweger syndrome, showing abnormal cerebral and cerebellar white matter, polymicrogyric-like cortex most severe in the perisylvian and perirolandic regions (asterisk), thin corpus callosum and hypoplasia of brainstem and cerebellar vermis.
A second illustration comes from a child born at 37 weeks gestation to healthy non-consanguineous parents, with a birth weight of 2.360kg, height of 46cm and head circumference of 30cm (-2.5SD). He had a sloping forehead, micrognathia, absent midpalmar creases, thick feet, and an inguinal hernia. Further evaluation revealed sensorineural hearing loss, optic nerve atrophy and retinal dystrophy. He had severe axial hypotonia and developed spastic quadriplegia. Focal seizures were diagnosed at age one month and were refractory to treatment. EEG showed a burst-suppression pattern. MRI at age one month showed a simplified gyral pattern with normal cortical thickness, predominantly over the anterior regions, bilateral perisylvian polymicrogyria as well as multiple subcortical heterotopia (Figure 3). Capillary zone electrophoresis and isoelectric focusing of serum transferrin revealed a type 1 pattern, pointing to a congenital disorder of glycosylation (CDG) due to a defect in N-glycan assembly. Analysis of dolichol-linked oligosaccharides was consistent with a defect in ALG11, and mutational analysis of ALG11 revealed compound heterozygosity for a missense c.479G>T (p.Gly160Val) and a splice site c.45-2A>T mutation[6].
Figure 3.Figure 3Brain MRI at age one month in a patient with compound heterozygous mutations in ALG11, showing a simplified gyral pattern predominantly over the anterior regions, multiple subcortical heterotopia (Panels A-C; stars), thin corpus callosum, hypoplasia of brainstem and cerebellar vermis, as well as a dysplastic cerebellar cortex (asterisk).
Both cases illustrate how metabolic investigations can assist with orienting further diagnostic work-up. Based on the combination of the clinical presentation and brain imaging, the differential diagnosis may include peroxisomal disorders screened for by very long chain fatty acids (VLCFA), congenital disorders of glycosylation screened for by isoelectric focusing of serum transferrins, as well as pyruvate and Krebs cycle defects or mitochondrial disorders screened for by lactate, pyruvate, glucose, amino acids, carnitine profile and ketone measurement in blood, and analysis of organic acids in urine.
Clinical Vignette 4
The proband presented at the age of two months with poor visual contact and axial hypotonia. She was born at 41 weeks gestation after an uneventful pregnancy and delivery with a birth weight of 2.660kg, height of 45.5cm and head circumference of 33cm. Transcranial ultrasound showed calcifications in the basal ganglia and white matter. Brain MRI at age six days showed multifocal polymicrogyria, white matter hyperintensity on T2-FLAIR, and cystic transformation of the white matter adjacent to the posterior horns of the lateral ventricles and temporal poles (Figure 4). CMV detection on a Guthrie card used for metabolic screening at age three days confirmed the presence of a congenital CMV infection. Fundoscopy was compatible with retinitis.
Figure 4.Figure 4Brain MRI at age six days in a patient with congenital cytomegalovirus infection, showing multifocal polymicrogyria (Panels A, B, D), cystic transformation of the white matter adjacent to the posterior horns of the lateral ventricles and temporal poles (Panels B-C; stars), as well as a dysplastic cerebellar cortex (Panel B; arrowhead).
Congenital infections including toxoplasma, rubella, CMV and herpes simplex, commonly abbreviated as TORCH, constitute a significant cause of MCD[7, 8]. Depending on the timing and severity of the infection, children with congenital infections often present with microcephaly, MCD (most commonly polymicrogyria), and variable degrees of cerebral and cerebellar white matter abnormalities, calcifications and cystic transformation. In addition, patients may show involvement of other organ systems, including the eyes, ears, and liver and kidney function. However, TORCH-infections can present with cortical malformations in the absence of any other brain or extra-CNS abnormalities[9]. Cystic transformation of the temporal poles should raise suspicion of congenital CMV infection[10]. Severe congenital microcephaly in combination with prominent subcortical calcifications with or without abnormalities of the corpus callosum, brainstem and cerebellum should include congenital Zika virus infection in its differential diagnosis[11, 12].
Clinical Vignette 5
This patient was born as the second child of healthy non-consanguineous parents after an uneventful pregnancy and delivery. She presented with hypotonia, bilateral cataracts and head circumference of 32 cm (-1.5SD). Transcranial ultrasound showed calcifications. Extensive TORCH-screening remained negative. At age 18 months, she developed focal seizures which were refractory to multiple anti-epileptic drugs. At the age of 30 months, she had a developmental age of 24 months for mental development and 17 months for motor development on the Bayley Scales for Infant Development II. Brain MRI at ages four months and three and a half years showed bilateral frontal polymicrogyria and extensive white matter hypersignal on T2-FLAIR sequences. There was a small porencephalic lesion with adjacent linear calcification in the left frontal lobe (Figure 5). The combination of eye abnormalities, MCD and white matter changes in a patient with no evidence of TORCH evoked a mutation in COL4A1 or COL4A2 as a potential differential diagnosis. Sanger sequencing confirmed the presence of a de novo c.3548G>T (p.Gly1183Val) missense mutation in COL4A1.
Figure 5.Figure 5Brain MRI at age three and a half years in a patient with a heterozygous mutation in COL4A1, showing bilateral frontal polymicrogyria (Panel A), a small porencephalic lesion with adjacent linear calcification in the left frontal lobe (Panel B), and extensive white matter hypersignal on T2-FLAIR sequences (Panel C).
This case illustrates that not all combinations of microcephaly, MCD, and intracerebral calcifications are the result of congenital infections, but can result from mutations in different genes, which together constitute the entity of ‘pseudo-TORCH’ syndromes.
Mutations in either COL4A1 or COL4A2 have been reported in association with a wide variety of brain malformations, ranging from focal PMG over pachygyria to porencephaly, multicystic encephalomalacia or hydranencephaly. In addition, intracerebral or intraventricular haemorrhage, microbleeds or calcifications are often present. In parallel to congenital infections, sensorineural hearing impairment, eye, heart, kidney or muscle abnormalities are frequent features[13, 14]. A further differential diagnosis to be evoked, in view of polymicrogyria in combination with band-like subcortical calcifications, are bi-allelic mutations in the OCLN gene which encode the tight junction protein occludin[15].
Further causes of pseudo-TORCH syndrome include mutations in JAM3 and USP18 genes, both associated with severe haemorrhagic brain destruction and poor prognosis[16, 17, 18].
Aicardi-Goutières syndrome, which is characterized by progressive cerebral atrophy, intracerebral calcifications affecting mainly the basal ganglia, and leukodystrophy, can result from mutations in multiple genes, including ADAR, IFIH1, TREX1, RNASEH2A, RNASEH2B, RNASEH2C and SAMHD1[19].
Clinical Vignette 6
The proband was a four-year-old boy, born at term after an uneventful pregnancy as the second child of non-consanguineous Caucasian parents. Birth parameters were normal with a head circumference of 34cm. He had unilateral renal pyelectasis and cryptorchidism. He presented at age three months with poor visual pursuit. He was found to have pale optic discs and abnormal visual evoked potentials. Brainstem evoked potentials were compatible with an auditory neuropathy. At age one year, he was unable to sit independently, he could reach for objects and grasp but could not yet manipulate, vocalizations were present but he did not use any words. At age two years, his weight was 10kg (-2.1SD), height was 80cm (-2.1SD) and head circumference was 43.6cm (-3.7SD). At that time, he developed focal seizures, characterized by behavioural arrest, pallor, sweating, nausea and vomiting. Brain MRI at ages seven, 14 and 26 months showed bilateral perisylvian polymicrogyria, global thinning of the white matter, left occipital gliosis, dysplastic basal ganglia, enlarged lateral ventricles, partial agenesis of the corpus callosum, fused colliculi, hypoplasia of the brainstem and cerebellar vermis, malrotation of the left hippocampus, and a multi-loculated pineal cyst (Figure 6). MCD gene panel analysis revealed a de novo c.5G>A (p.Arg2His) mutation in TUBA1A, which has been reported earlier in another family [3].
Figure 6.Figure 6Brain MRI at age 26 months in a patient with heterozygous mutation in TUBA1A, showing bilateral perisylvian polymicrogyria (Panels A and C; asterisk), global thinning of the white matter, right occipital gliosis (Panel D; star), dysplastic basal ganglia (Panels A and C), enlarged lateral ventricles with hooked aspect of the anterior horns, partial agenesis of the corpus callosum (Panel B), and hypoplasia of the brainstem and cerebellar vermis (Panel B), as well as dysgenesis of cerebellar vermis (Panel D, arrow).
Mutations in either of the genes belonging to the tubulin superfamily, TUBA1A, TUBB2A, TUBB2B, TUBB3, TUBB5, or TUBG1, have been associated with MCD. These malformations are collectively referred to as tubulinopathies [20, 21]. Mutations in some of these genes are associated with a polymicrogyria-like cortical malformation, which has been termed tubulin-related dysgyria [22]. The tubulin isotypes are highly expressed in the foetal and adult brain and nervous system and are required for normal microtubule function [23]. Microtubules, heterodimeric polymers of alpha and beta tubulins, are necessary for a range of intracellular processes involved in neurogenesis, neuronal migration and axon growth [24, 25]. Mutations in the tubulin genes are often associated with a combination of brain malformations, involving the cerebral cortex, basal ganglia, corpus callosum, brainstem and cerebellum. Basal ganglia are often dysplastic due to dysgenesis of the anterior limb of the internal capsule resulting in a hooked aspect of the frontal horns of the lateral ventricles (Figure 6, A and C). Callosal abnormalities range from hypoplasia over partial to complete agenesia. There is frequent hypoplasia of brainstem and cerebellum with cerebellar vermis dysgenesis (Figure 6, D) [26]. The degree of cortical malformations is highly variable, can range from polymicrogyria to lissencephaly, and is commonly referred to as ‘tubulin-related dysgyria’[22, 26]. The imaging features associated with the tubulinopathies illustrate how a specific MRI-pattern may sometimes be of great value for the orientation of the molecular genetic work-up.
Before proceeding to NGS, microarray-based comparative genomic hybridisation (array CGH) is indicated to rule out copy number variants as a potential cause of MCD[27, 28].
Further molecular genetic work-up may be oriented based on all of the above. Depending on the health-care system and resources of a specific country, work-up may vary from targeted gene sequencing by Sanger, over parallel sequencing using targeted gene panels, to whole exome or whole genome sequencing.
Finding mutations in genes known to be associated with MCD may result in a further delineation of the MCD-phenotype, as illustrated by the recent publications in the field of tubulinopathies [29, 30]. In addition, the use of whole exome or genome sequencing may also facilitate the identification of novel genes involved in brain development, as illustrated for example by the identification of mutations in INTS8[31] or COL3A1[32].
Recent evolutions in the field of MCD and outlook for the future
1. The mTOR pathway is everywhere
Clinical Vignette 7
The proband was born at 39 weeks gestation after an uneventful pregnancy without high risk follow-up. On the third day of life, focal seizures were noticed. EEG showed a burst-suppression pattern. Brain MRI on day 12 showed right hemimegalencephaly with polymicrogyria and nodular heterotopia lining the right lateral ventricle (Figure 7). Head circumference at 25 days of life was 39.3cm (Pc 83, +1SD). Seizures were refractory to medical treatment and he was referred for epilepsy surgery. He has severe developmental delay, left hemiparesis and has been tube-fed since the age of 15 months. He has a pigmented skin lesion on the right side of his forehead. A gene panel for MCD including genes encoding mTOR pathway proteins was first performed in his blood and was negative. Subsequently, the gene panel was tested with DNA extracted from skin fibroblasts obtained from the forehead lesion and a c.1624G>A, p.(Glu542Lys) pathogenic variant in the PIK3CA gene was detected in 30% of cells. The mutation was not detected by Sanger sequencing of DNA obtained from a buccal swab.
This case illustrates how somatic or germline pathogenic variants in genes encoding proteins involved in the mTOR signalling pathway can underlie the development of various MCD, including focal cortical dysplasia, tuberous sclerosis-associated cortical tubers, megalencephaly with or without polymicrogyria, and hemimegalencephaly.
Figure 7.Figure 7Brain MRI on day 12 showed right hemimegalencephaly with polymicrogyria (Panels A and B). A heterozygous pathogenic variant in PIK3CA was identified in 30% of skin fibroblasts derived from the epidermal nevus on the forehead.
In 2002, the link between the TSC1 and TSC2 gene products hamartin and tuberin and the mTOR-pathway was established[33, 34, 35]. This formed the basis for the first clinical trials with mTOR-inhibitors for the treatment of TSC-related features, including subependymal giant cell astrocytoma, angiomyolipoma and epilepsy[36, 37, 38]. More recently, mutations in mTOR, and in PIK3CA, PIK3R2, PTEN, AKT and CCND2 acting upstream of mTOR, have been identified in individuals with a wide variety of MCD including megalencephaly with or without polymicrogyria, capillary malformation, polydactyly or hydrocephalus (MCAP, MPPH), hemimegalencephaly, and focal cortical dysplasia[39, 40, 41, 42, 43]. Most patients with mutations in the PI3K/AKT/mTOR pathway have megalencephaly, although this is not an obligatory feature[44].
The mTOR pathway has also blurred the borders between focal cortical dysplasia and focal epilepsies. Mutations in DEPDC5, NPRL2 and NPRL3 encoding components of the Gap Activity TOward Rags 1 (GATOR1) complex, involved in the inhibition of the mTOR complex 1 (mTORC1), have been identified in familial cases of focal cortical dysplasia, as well as in families where some individuals have focal epilepsy with focal cortical dysplasia on MRI, and others have focal epilepsy with apparently normal MRI[45, 46, 47]. The occurrence of non-penetrance of mutations in these genes represents an additional challenge for genetic counselling[47].
2. Somatic mosaicism and single-neuron sequencing
Somatic mutations are increasingly identified in neurodevelopmental diseases. Somatic mutations can arise during the course of prenatal brain development and cause neurological disease, even when present at low levels of mosaicism[48, 49]. Somatic mutations have been detected in a wide variety of genes associated with MCD, including LIS1, DCX, FLNA, TUBB2B, as well as the genes encoding actors of the mTOR-pathway[42, 48]. The patterns in which somatic mutations distribute in the human brain are starting to be unraveled. Using high-coverage whole-genome sequencing of single neurons from a normal individual has enabled identification of spontaneous somatic mutations as clonal marks to track cell lineages in the human brain[50]. The rapid development of single-cell RNA sequencing further facilitates the use of genome-wide gene expression patterns to assist with the characterization of cortical neurons, and provides new insights into the transitions that establish neuronal identity during development, differentiation, activity, and disease[51].
3. After tubulinopathies - dyneinopathies
When a patient presents with a brain malformation in combination with signs of axonal neuropathy, spinal muscular atrophy and/or Arthrogryposis Multiplex Congenita (AMC), a dyneinopathy due to a heterozygous mutation in DYNC1H1 or BICD2 should be suspected[52, 53, 54]. Brain malformations, if present, include posterior pachygyria, frontal polymicrogyria or nodular heterotopia. The corpus callosum can be either thickened or hypoplastic. Basal ganglia can be dysmorphic. Hypoplasia of brainstem and cerebellar vermis is possible. Patients can be normo- or microcephalic. The phenotypic spectrum is very wide, with age at presentation ranging from the prenatal period to adulthood, and variable degrees of intellectual disability, motor impairment and seizures[52, 54]. Although dyneinopathies may combine brain malformations with muscle involvement, they clearly differ from the muscle-eye-brain spectrum of malformations which are characterized by cobblestone malformation and muscular dystrophy.
4. The brain and cilia – more than Joubert syndrome alone
Ciliopathies are a group of genetic disorders caused by defects in primary ciliary structure and/or function. Ciliopathies are characterized by pleiotropic clinical features, with mutations leading to distinctive developmental and/or degenerative phenotypes in several tissues, including the retina, kidney and nervous system. Nervous system-related defects include neural tube, migration and cerebellar development, retinal degeneration, anosmia and obesity. This plethora of nervous system effects suggests many potential roles of cilia in development and function[55, 56]. Primary cilia are now recognized as key integrators of extracellular ligand-based signaling and cellular polarity, which regulate neuronal cell fate, migration differentiation, as well as a host of adult behaviours.
The identification of bi-allelic mutations in RTTN, the gene encoding rotatin, in individuals with brain malformations encompassing polymicrogyria, pachygyria with or without associated heterotopia or CNS cysts sheds further light on the link between primary cilia and the developing cerebral cortex[57, 58, 59]. Further phenotypic characterisation, identification of causative genes and research focusing on gene function will provide additional insights that link ciliary function with key developmental signaling pathways[55].
5. COST Action CA16118 Neuro-MIG
Multidisciplinary MCD teams are not common in most medical centers, and neuroscientists or biophysicists studying cortical development and advanced neuroimaging are not always connected to clinicians. As a result, expertise on MCD is very fragmented, confined to the personal interest of a few experts and access to state of the art medical care is unequally distributed. The consequence is that patients and families spend a long time searching for answers to questions about causes, prognosis, and management.
In order to tackle these problems, a multidisciplinary network on brain malformations, Neuro-MIG, has been created and recently received support from the European Community through a COST Action grant (http://www.cost.eu/COST_Actions/ca/CA16118; http://neuro-MIG.org). The Neuro-MIG consortium kicked off in the spring of 2017 and brings together researchers and clinicians from various disciplines to create a pan-European MCD network. Its aim is to advance the understanding of MCD and utilise this knowledge to improve diagnosis and clinical management. In particular, the network goals are 1) to harmonise MCD classification in order to develop guidelines for clinical management, 2) to create best practice diagnostic pipelines which can be uniformly adopted in Europe, 3) to coordinate databases in different countries and allow epidemiology studies, 4) to identify common pathophysiological mechanisms, which allows grouping of disorders and exploration of future common therapeutic approaches by stimulating translational and transnational exchange and research, 5) to create a web-based platform for case and MRI review, 6) to educate young clinicians and scientists, 7) to join research initiatives aimed at developing appropriate treatments, 8) to disseminate results and promote public awareness. This international and interdisciplinary collaborative effort creates a driving force to move the field forward with the ultimate goal of improving the lives of patients with MCD and their families.
Acknowledgements
This John Stobo Prichard Award is dedicated to:
• The patients and families – for allowing me to be part of their journey;
• Linda – for believing in me, for allowing me to follow my passion;
• Danièle and Nathalie and the team at UZ Brussel – for looking after my patients when I am away, for being the cornerstones on which I can build;
• The VUB community – for daring to be ‘radical dreamers: radically digital, radically sustainable, radically humane and radically diverse (Caroline Pauwels, Rector)’
• Eva and Fred and my Montreal family – for introducing me to the joys of research and international collaboration, for making me grow beyond my dreams;
• My EPISTOP and Neuro-MIG families – for sharing my enthusiasm, for being great colleagues and friends;
• Rick and my Melbourne family – for providing me with a home away from home to think, write, cross-fertilise;
• Petrus – for operatic interludes and precious friendship;
• Waney – for your courage, critical mind, and wonderful company;
• The King Baudouin Foundation team – for striving for a better society and having me on board;
• Hendrik, Olivia and Rosalie – my treasures;
• Erik – my generous and supportive partner.
This manuscript is based on the John Stobo Prichard Award lecture entitled ’Developmental Brain Malformations – of genes and pathways‘ presented at the 14th International Child Neurology Congress in 2016 in Amsterdam, the Netherlands.
Funding
AJ is supported by the Research Foundation – Flanders (FWO), the Willy Gepts Scientific Fund and the VUB Research Foundation.
Conflict of interest
The author is not aware of any financial or non-financial competing interests which affect the content of this report.
Author’s contributions
AJ contributed through data collection, interpretation and manuscript revisions. EI was primarily involved in the development of the manuscript as well as data interpretation.
This is an Open Access article distributed under the terms of the Creative Commons Attribution License (http://creativecommons.org/licenses/by/4.0), which permits unrestricted use, distribution, and reproduction in any medium, provided the original work is properly credited. The Creative Commons Public Domain Dedication waiver (http://creativecommons.org/publicdomain/zero/1.0/) applies to the data made available in this article, unless otherwise stated.
References
- Barkovich AJ, Guerrini R, Kuzniecky RI, Jackson GD, Dobyns WB. A developmental and genetic classification for malformations of cortical development: update 2012.. Brain. 2012; 135((Pt 5)):1348-69. PubMed
- Sawyer SL, Hartley T, Dyment DA, Beaulieu CL, Schwartzentruber J, Smith A, et al. . Utility of whole-exome sequencing for those near the end of the diagnostic odyssey: time to address gaps in care.. Clin Genet. 2016; 89(3):275-84. PubMed
- Srivastava S, Cohen JS, Vernon H, Baranano K, McClellan R, Jamal L, et al. . Clinical whole exome sequencing in child neurology practice.. Ann Neurol. 2014; 76(4):473-83. PubMed
- Vissers L, van Nimwegen KJM, Schieving JH, Kamsteeg EJ, Kleefstra T, Yntema HG, et al. . A clinical utility study of exome sequencing versus conventional genetic testing in pediatric neurology.. Genet Med. 2017; 19(9):1055-63. PubMed
- Handley MT, Morris-Rosendahl DJ, Brown S, Macdonald F, Hardy C, Bem D, et al . Mutation spectrum in RAB3GAP1, RAB3GAP2, and RAB18 and genotype-phenotype correlations in Warburg Micro syndrome and Martsolf syndrome.. Hum Mutat. 2013; 34(5):686-96. PubMed
- Regal L, van Hasselt PM, Foulquier F, Cuppen I, Prinsen H, Jansen K, et al. . ALG11-CDG: Three novel mutations and further characterization of the phenotype.. Mol Genet Metab Rep.. 2015; 2( ):16-9. PubMed
- Devakumar D, Bamford A, Ferreira MU, Broad J, Rosch RE, Groce N, et al . Infectious causes of microcephaly: epidemiology, pathogenesis, diagnosis, and management.. Lancet Infect Dis.. 2018; 18(1):e1-e13. PubMed
- Teissier N, Fallet-Bianco C, Delezoide AL, Laquerriere A, Marcorelles P, Khung-Savatovsky S, et al. . Cytomegalovirus-induced brain malformations in fetuses.. J Neuropathol Exp Neurol.. 2014; 73(2):143-58. PubMed
- Jansen AC, Robitaille Y, Honavar M, Mullatti N, Leventer RJ, Andermann E, et al. . The histopathology of polymicrogyria: a series of 71 brain autopsy studies.. Dev Med Child Neurol.. 2016; 58(1):39-48. PubMed
- van der Knaap MS, Vermeulen G, Barkhof F, Hart AA, Loeber JG, Weel JF. Pattern of white matter abnormalities at MR imaging: use of polymerase chain reaction testing of Guthrie cards to link pattern with congenital cytomegalovirus infection.. Radiology. 2004; 230(2):529-36. PubMed
- Krauer F, Riesen M, Reveiz L, Oladapo OT, Martinez-Vega R, Porgo TV, et al. . Zika Virus Infection as a Cause of Congenital Brain Abnormalities and Guillain-Barré Syndrome: Systematic Review.. PLoS Med.. 2017; 14(1):e1002203. PubMed
- Russo FB, Jungmann P, Beltrao-Braga PCB. Zika infection and the development of neurological defects.. Cell Microbiol.. 2017; 19(6)PubMed
- Meuwissen ME, de Vries LS, Verbeek HA, Lequin MH, Govaert PP, Schot R, et al. . Sporadic COL4A1 mutations with extensive prenatal porencephaly resembling hydranencephaly.. Neurology. 2011; 76(9):844-6. PubMed
- Meuwissen ME, Halley DJ, Smit LS, Lequin MH, Cobben JM, de Coo R, et al. . The expanding phenotype of COL4A1 and COL4A2 mutations: clinical data on 13 newly identified families and a review of the literature.. Genet Med.. 2015; 17(11):843-53. PubMed
- O'Driscoll MC, Daly SB, Urquhart JE, Black GC, Pilz DT, Brockmann K, et al. . Recessive mutations in the gene encoding the tight junction protein occludin cause band-like calcification with simplified gyration and polymicrogyria.. Am J Hum Genet.. 2010; 87(3):354-64. PubMed
- Akawi NA, Canpolat FE, White SM, Quilis-Esquerra J, Morales SM, Gamundi MJ, et al. . Delineation of the clinical, molecular and cellular aspects of novel JAM3 mutations underlying the autosomal recessive hemorrhagic destruction of the brain, subependymal calcification, and congenital cataracts.. Hum Mutat.. 2013; 34(3):498-505. PubMed
- Meuwissen ME, Schot R, Buta S, Oudesluijs G, Tinschert S, Speer SD, et al. . Human USP18 deficiency underlies type 1 interferonopathy leading to severe pseudo-TORCH syndrome.. J Exp Med.. 2016; 213(7):1163-74. PubMed
- Mochida GH, Ganesh VS, Felie JM, Gleason D, Hill RS, Clapham KR, et al. . A homozygous mutation in the tight-junction protein JAM3 causes hemorrhagic destruction of the brain, subependymal calcification, and congenital cataracts.. Am J Hum Genet. 2010; 87(6):882-9. PubMed
- Crow YJ, Pagon RA, Adam MP, Ardinger HH, Wallace SE, Amemiya A, Bean LJH, et al. . Aicardi-Goutieres Syndrome.. GeneReviews(R). Seattle (WA). 1993. PubMed
- Bahi-Buisson N, Poirier K, Fourniol F, Saillour Y, Valence S, Lebrun N, et al. . The wide spectrum of tubulinopathies: what are the key features for the diagnosis?. Brain.. 2014; 137(Pt 6):1676-700. PubMed
- Cushion TD, Dobyns WB, Mullins JG, Stoodley N, Chung SK, Fry AE, et al. . Overlapping cortical malformations and mutations in TUBB2B and TUBA1A.. Brain.. 2013; 136(Pt 2):536-48. PubMed
- Bahi-Buisson N, Poirier K, Fourniol F, Saillour Y, Valence S, Lebrun N, et al. . The wide spectrum of tubulinopathies: what are the key features for the diagnosis?. Brain.. 2014; 137(Pt 6):1676-700. PubMed
- Leandro-García LJ, Leskelä S, Landa I, Montero-Conde C, López-Jiménez E, Letón R, et al. . Tumoral and tissue-specific expression of the major human beta-tubulin isotypes.. Cytoskeleton (Hoboken).. 2010; 67(4):214-23. PubMed
- Tischfield MA, Engle EC. Distinct alpha- and beta-tubulin isotypes are required for the positioning, differentiation and survival of neurons: new support for the ‘multi-tubulin’ hypothesis.. Biosci Rep.. 2010; 30(5):319-30. PubMed
- Suri C, Hendrickson TW, Joshi HC, Naik PK. Molecular insight into γ-γ tubulin lateral interactions within the γ-tubulin ring complex (γ-TuRC).. J Comput Aided Mol Des.. 2014; 28(9):961-72. PubMed
- Oegema R, Cushion TD, Phelps IG, Chung SK, Dempsey JC, Collins S, et al. . Recognizable cerebellar dysplasia associated with mutations in multiple tubulin genes.. Hum Mol Genet.. 2015; 24(18):5313-25. PubMed
- Jansen A, Andermann E. Genetics of the polymicrogyria syndromes. J Med Genet. 2005; 42(5):369-78. PubMed
- Kariminejad R, Lind-Thomsen A, Tumer Z, Erdogan F, Ropers HH, Tommerup N, et al. . High frequency of rare copy number variants affecting functionally related genes in patients with structural brain malformations.. Hum Mutat.. 2011; 32(12):1427-35. PubMed
- Amrom D, Tanyalcin I, Verhelst H, Deconinck N, Brouhard GJ, Decarie JC, et al. . Polymicrogyria with dysmorphic basal ganglia? Think tubulin!. Clin Genet.. 2014; 85(2):178-83. PubMed
- Jansen AC, Oostra A, Desprechins B, De Vlaeminck Y, Verhelst H, Regal L, et al. . TUBA1A mutations: from isolated lissencephaly to familial polymicrogyria.. Neurology. 2011; 76(11):988-92. PubMed
- Oegema R, Baillat D, Schot R, van Unen LM, Brooks A, Kia SK, et al. . Human mutations in integrator complex subunits link transcriptome integrity to brain development.. PLoS Genet.. 2017; 13(5):e1006809. PubMed
- Vandervore L, Stouffs K, Tanyalcin I, Vanderhasselt T, Roelens F, Holder-Espinasse M, et al. . Bi-allelic variants in COL3A1 encoding the ligand to GPR56 are associated with cobblestone-like cortical malformation, white matter changes and cerebellar cysts.. J Med Genet.. 2017; 54(6):432-40. PubMed
- Gao X, Zhang Y, Arrazola P, Hino O, Kobayashi T, Yeung RS, et al. . TSC tumour suppressor proteins antagonize amino-acid-TOR signalling.. Nat Cell Biol.. 2002; 4(9):699-704. PubMed
- Inoki K, Li Y, Zhu T, Wu J, Guan KL. TSC2 is phosphorylated and inhibited by Akt and suppresses mTOR signalling.. Nat Cell Biol.. 2002; 4(9):648-57. PubMed
- Tee AR, Fingar DC, Manning BD, Kwiatkowski DJ, Cantley LC, Blenis J. Tuberous sclerosis complex-1 and -2 gene products function together to inhibit mammalian target of rapamycin (mTOR)-mediated downstream signaling.. Proc Natl Acad Sci U S A. 2002; 99(21):13571-6. PubMed
- Bissler JJ, Kingswood JC, Radzikowska E, Zonnenberg BA, Frost M, Belousova E, et al. . Everolimus for angiomyolipoma associated with tuberous sclerosis complex or sporadic lymphangioleiomyomatosis (EXIST-2): a multicentre, randomised, double-blind, placebo-controlled trial.. Lancet. 2013; 381(9869):817-24. PubMed
- Franz DN, Belousova E, Sparagana S, Bebin EM, Frost M, Kuperman R, et al. . Efficacy and safety of everolimus for subependymal giant cell astrocytomas associated with tuberous sclerosis complex (EXIST-1): a multicentre, randomised, placebo-controlled phase 3 trial.. Lancet. 2013; 381(9861):125-32. PubMed
- French JA, Lawson JA, Yapici Z, Ikeda H, Polster T, Nabbout R, et al. . Adjunctive everolimus therapy for treatment-resistant focal-onset seizures associated with tuberous sclerosis (EXIST-3): a phase 3, randomised, double-blind, placebo-controlled study.. Lancet.. 2016; 388(10056):2153-63. PubMed
- D'Gama AM, Geng Y, Couto JA, Martin B, Boyle EA, LaCoursiere CM, et al. . Mammalian target of rapamycin pathway mutations cause hemimegalencephaly and focal cortical dysplasia.. Ann Neurol.. 2015; 77(4):720-5. PubMed
- Jansen LA, Mirzaa GM, Ishak GE, O'Roak BJ, Hiatt JB, Roden WH, et al. . PI3K/AKT pathway mutations cause a spectrum of brain malformations from megalencephaly to focal cortical dysplasia.. Brain.. 2015; 138(Pt 6):1613-28. PubMed
- Mirzaa G, Parry DA, Fry AE, Giamanco KA, Schwartzentruber J, Vanstone M, et al. . De novo CCND2 mutations leading to stabilization of cyclin D2 cause megalencephaly-polymicrogyria-polydactyly-hydrocephalus syndrome.. Nat Genet.. 2014; 46(5):510-5. PubMed
- Mirzaa GM, Riviere JB, Dobyns WB. Megalencephaly syndromes and activating mutations in the PI3K-AKT pathway: MPPH and MCAP.. Am J Med Genet C Semin Med Genet.. 2013; 163C(2):122-30. PubMed
- Vahidnezhad H, Youssefian L, Uitto J. Klippel-Trenaunay syndrome belongs to the PIK3CA-related overgrowth spectrum (PROS).. Exp Dermatol.. 2016; 25(1):17-9. PubMed
- Mirzaa GM, Conti V, Timms AE, Smyser CD, Ahmed S, Carter M, et al. . Characterisation of mutations of the phosphoinositide-3-kinase regulatory subunit, PIK3R2, in perisylvian polymicrogyria: a next-generation sequencing study.. Lancet Neurol.. 2015; 14(12):1182-95. PubMed
- Leventer RJ, Jansen A, Pilz DT, Stoodley N, Marini C, Dubeau F, et al. . Clinical and imaging heterogeneity of polymicrogyria: a study of 328 patients.. Brain.. 2010; 133(Pt 5):1415-27. PubMed
- Ricos MG, Hodgson BL, Pippucci T, Saidin A, Ong YS, Heron SE, et al. . Mutations in the mammalian target of rapamycin pathway regulators NPRL2 and NPRL3 cause focal epilepsy.. Ann Neurol.. 2016; 79(1):120-31. PubMed
- Scheffer IE, Heron SE, Regan BM, Mandelstam S, Crompton DE, Hodgson BL, et al. . Mutations in mammalian target of rapamycin regulator DEPDC5 cause focal epilepsy with brain malformations.. Ann Neurol.. 2014; 75(5):782-7. PubMed
- Jamuar SS, Lam AT, Kircher M, D'Gama AM, Wang J, Barry BJ, et al. . Somatic mutations in cerebral cortical malformations.. N Engl J Med.. 2014; 371(8):733-43. PubMed
- Poduri A, Evrony GD, Cai X, Walsh CA. Somatic mutation, genomic variation, and neurological disease.. Science. 2013; 341(6141):1237758. PubMed
- Evrony GD, Lee E, Mehta BK, Benjamini Y, Johnson RM, Cai X, et al. . Cell lineage analysis in human brain using endogenous retroelements.. Neuron.. 2015; 85(1):49-59. PubMed
- Johnson MB, Walsh CA. Cerebral cortical neuron diversity and development at single-cell resolution.. Curr Opin Neurobiol.. 2017; 42(9):16- . PubMed
- Laquerriere A, Maillard C, Cavallin M, Chapon F, Marguet F, Molin A, et al. . Neuropathological Hallmarks of Brain Malformations in Extreme Phenotypes Related to DYNC1H1 Mutations.. J Neuropathol Exp Neurol.. 2017; 76(3):195-205. PubMed
- Peeters K, Bervoets S, Chamova T, Litvinenko I, De Vriendt E, Bichev S, et al. . Novel mutations in the DYNC1H1 tail domain refine the genetic and clinical spectrum of dyneinopathies.. Hum Mutat.. 2015; 36(3):287-91. PubMed
- Poirier K, Lebrun N, Broix L, Tian G, Saillour Y, Boscheron C, et al. . Mutations in TUBG1, DYNC1H1, KIF5C and KIF2A cause malformations of cortical development and microcephaly.. Nat Genet.. 2013; 45(6):639-47. PubMed
- Guemez-Gamboa A, Coufal NG, Gleeson JG. Primary cilia in the developing and mature brain.. Neuron.. 2014; 82(3):511-21. PubMed
- Guo J, Higginbotham H, Li J, Nichols J, Hirt J, Ghukasyan V, et al. . Developmental disruptions underlying brain abnormalities in ciliopathies.. Nat Commun.. 2015; 6:7857. PubMed
- Grandone A, Torella A, Santoro C, Giugliano T, Del Vecchio Blanco F, Mutarelli M, et al. . Expanding the phenotype of RTTN variations: a new family with primary microcephaly, severe growth failure, brain malformations and dermatitis.. Clin Genet.. 2016; 90(5):445-50. PubMed
- Kheradmand KS, Verbeek E, Engelen E, Schot R, Poot RA, de Coo IF, et al. . RTTN mutations link primary cilia function to organization of the human cerebral cortex.. Am J Hum Genet.. 2012; 91(3):533-40. PubMed
- Shamseldin H, Alazami AM, Manning M, Hashem A, Caluseiu O, Tabarki B, et al. . RTTN Mutations Cause Primary Microcephaly and Primordial Dwarfism in Humans.. Am J Hum Genet.. 2015; 97(6):862-8. PubMed
Main Article Content
Abstract
Malformations of cortical development (MCD) constitute a group of brain disorders which are mainly genetic in origin. MCD form an important cause of cerebral palsy, intellectual disability and refractory epilepsy. Despite considerable progress which has been facilitated by advances in the fields of neuroimaging and genetics, the high degree of phenotypic and genotypic heterogeneity associated with MCD continues to hamper etiological diagnosis and cousneling of numerous patients and families.
The first section of this manuscript is a plea for detailed clinical phenotyping in MCD and reviews the differential diagnosis and clinical work-up based on six clinical case reports. The second part provides a review of personal highlights in the field of MCD-research, and ends with an outlook to the joint efforts of international and interdisciplinary collaborations, which will hopefully result in better care for patients with MCD and their families.
Article Details

This work is licensed under a Creative Commons Attribution-NonCommercial-ShareAlike 4.0 International License.